The current context of floating offshore wind energy
The floating offshore wind (FOW) faces several challenges that lock their massive deployment, such as the need to improve the reliability of floating platforms, to facilitate their mass serial production, and to reduce their LCoE (higher capacity factors, reduced CAPEX and OPEX, lower insurance rate…).
Because of their precommercial status, current FOW platform prototypes are overdesigned, and undersized (currently installed FOW units’ maximum nominal power is <10MW, in a water depth between 260 and 300 m, not allowing for the full exploitation of all offshore wind energy potential) and not cost or mass optimized. Current FOW
platform designs need to tackle several challenges to become a fully commercial reality for wind farms with bigger WTs.
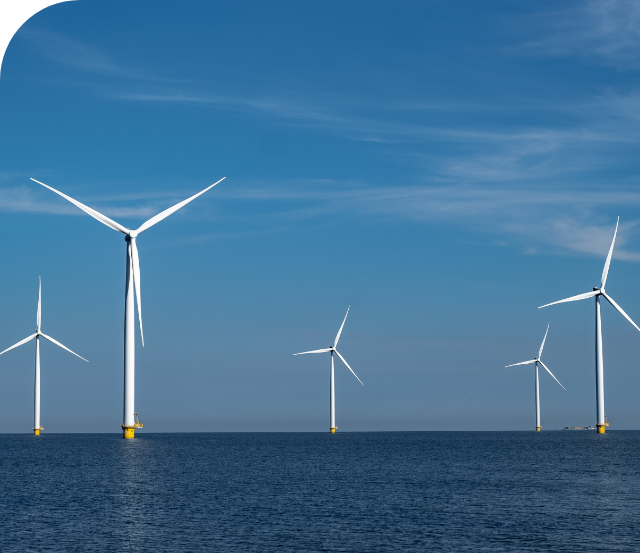
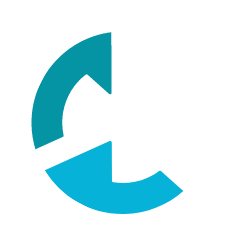
Innovation for the future of floating offshore wind energy
Challenge
The floating offshore wind (FOW) faces several challenges that lock their massive deployment, such as the need to improve the reliability of floating platforms, to facilitate their mass serial production, and to reduce their LCoE (higher capacity factors, reduced CAPEX and OPEX, lower insurance rate…).
Current FOW platform designs need to become a fully commercial reality for wind farms with bigger WTs. On the other hand, many offshore wind farms (WF) will reach their end of life in the coming years, calling attention to the importance “circularity by design” to optimise (critical) raw material use.
Solution
MADE4WIND will develop and test innovative components concepts for a 15 MW FWT consisting of new designs and manufacturing techniques for blades, substructure, and drivetrain.
These innovations will jointly allow future FWT to include new circular lightweighted materials, minimize the impact of sea habitats, increase operational availability, reduce maintenance needs and minimize LCoE; thus, unlocking the massive deployment of more than 15 MW floating WFs in Europe and worldwide.
Impacts
MADEmaximise the deployment of renewables and the use of electricity to fully decarbonise Europe’s energy supply. In addition, European Green Deal aims to transform the EU into a fair and prosperous society, with a modern, resource-efficient and competitive economy where there are no net emissions of greenhouse gases in 2050 and where economic growth is decoupled from resource use.
4WIND will contribute to
Technical objectives to address the most relevant TECHNOLOGICAL CHALLENGES
– Address the lightweight and smart design of WT blades through new blade toughening material solutions improving the structural integrity at critical design details enabling lower blade mass.
– Explore composite preform solutions enabling reduced blade production costs and improved factory throughput (modular manufacturing), while reducing manufacturing defects and improving the reliability of large WTB.
– Develop and test innovative composite recycling solutions for the WT blades, enabling blade circularity with minimized waste and cost at decommissioning, yet reducing the need for composite raw materials
– Develop and test innovative circular concrete materials solutions (R4) for the floater and anchor, enabling reduced environmental impacts with a lower CO2 footprint, ensuring structural integrity in a marine environment, and improving material durability through corrosion and biofouling resistance.
– Design Lightweight floater concept with reduced production & installation costs, easy to cast and transport.
– Optimize the structural and weight requirements of the gravity anchor enabling smaller dimensions.
– Explore the use of Aluminium rebar as a substitute to steel enabling increased floater lifetime by reducing corrosion risk, potentially reducing the thickness layer of the concrete shell, and allowing the use of new cement
mix with reduced CO2 footprint.
– Optimize the mooring systems by replacing heavy mooring chains with lighter synthetic tendons (R8) enabling to decrease their weight.
– Implement a numerical methodology for modelling of reinforced concrete in the structural analyses enabling optimized TLP floating component design
– Develop a compact, optimized and lightweight, high power density drivetrain in order to obtain the most cost-effective option for large floating offshore wind turbines, also minimising the use of rare earth material.
– Improved generator technology by evaluating main technological parameters (characteristics, efficiency, weight…) in order to obtain optimum ratio power/speed.
– Innovative reliable converter design based on advanced semiconductor technologies to obtain an optimal solution considering mechanical loads, power losses, cost, and LCoE reduction.
– Upgrade the electrical drivetrain reliability by: (1) Designing a new main power module and (2) reducing the total number of elements used in the electrical system; and also (3) developing new switching and parallelization
strategies and advanced control algorithms, and (4) introducing a novel monitoring system
– Develop an improved cost model (R14) to account for the components’ materials, design, and development costs enabling faster evaluation of various design strategies.
– Develop a virtual floating 15MW WT (R15) using Siemens SG 14-236 prototype (combined with a publicly available WT i.e. IEA 15 MW) to establish the impact of improved MADE4WIND components (blade, drivetrain, floater, Tandon, and anchor) on the WT cost and WF LCoE.
– Utilize in-house state-of-the-art simulation tools (SIMA and DIWA1) to improve the understanding of the offshore FWTs and WFs related physical phenomena, which is a necessity to optimize the design of future large-scale FWTs (≥15MW)/FWFs (Floating Wind Farms) according to technical, economic and environmental demands.
– Validate the different critical components (blades, drivetrain, support floating structure) in relevant conditions in order to achieve high durability, decreased maintenance, and reduced failure rates.
– Ensure a high level of techno-economic feasibility while ensuring high availability of system operation offshore to achieve optimised LCoE of the system. 1 Panjwani et al. Effect of Wake Meandering on Aeroelastic Response of a Wind Turbine Placed in a Park, Journal of Physics: Conference Series.
– Validate the numerical models developed by advanced lab testing in order to achieve a high level of accuracy of the simulation framework allowing a safe reduction in the over-engineering of devices’ specifications.
– Biodiversity assessments and biodiversity protection strategy complementing existing mandatory Environmental Impact Assessment (EIA) will minimise potential impacts on protected species and habitats.
– Life Cycle Assessment (LCA) will ensure that targeted GHG savings are achieved throughout the supply chain and no significant harm is done to marine ecosystem or any other planetary boundary in addition to the climate.
– A maximized contribution to the objectives of the Mission Healthy Oceans by-design, aiming to integrate with the maritime economy promoting circular, low-carbon, multi-purpose use of marine space.
– Reduced wind turbine cost thanks to smart blade manufacturing, lighter and efficient drivetrains, and optimized support structure components (light weight floater, anchors, synthetic tendons).
– Reduced Levelized Cost of Energy (LCoE) to around 75 €/MWh @ 7% discount rate and 55 €/MWh @ 3% discount rate, making offshore FWF a competitive renewable energy (in line with SET Plan targets for offshore wind2, e.g. 62-106 €/MWh for floating).
– Reducing investment risk thanks to monitoring and predictive computational tools (R14) for ensuring structural integrity to reduce failure and to increase installability and maintainability.
– Social sustainability assessment including social LCA (sLCA) to identify critical risks early on.
– (Critical) raw materials assessment to reduce EU supply chain dependencies and preserve resources for future
generations.
– A potential deployment map will show competition, ecological, and technological constraints.
– Geographic Information System (GIS) maps consisting of noise and biodiversity for few EU offshore sites.
– Identify stakeholders involved in the key Offshore Wind Energy initiatives promoted by WIND Energy Europe, ETIP SNET and consider their needs in designing a compatible solution that receives public acceptance.
– Creating new businesses and job opportunities for on-site shore assembly, transport, installation, O&M.
Achieve market up-take by establishing: a global plan for dissemination and exploitation of results, supported by dissemination in journals, events and clustering activities interacting with end users and wind stakeholders. The exploitation and further business plans will include specific Offshore Wind
Energy market complexity, IPR, regulatory framework, market up-take barriers, analysis of the social perception of technological results and fostering the massive replication into the offshore wind sector. To this end, MADE4WIND will take maximum advantage of the existing knowledge on relevant previous R&D projects
THE 4 PILLARS OF MADE4WIND
Lightweight, recyclable, and easy to manufacture WT blades
New toughening materials for wind turbine blades.
MADE4WIND will go beyond SoA by exploring, maturing, and tailoring the toughening
technology based on interleaves, made of thin, lightweight (20-50 g/m2), cost-effective microfiber polymeric layers, for use in WT blades. We will make use of the patented7 manufacturing process of graded microfibre nonwoven interleaves by FIBERTEX, which in pilot tests by AAU have shown to increase the fracture toughness in conventional
blade laminates by ~50%8. SoA experimental tests9 will be further developed to investigate the toughening effect of embedded interleaves. Advanced simulation methods8 will be enhanced and the interleave toughening technology will be optimized by improving the microfibre distribution and through-the-thickness material grading.
Preform technology for modular manufacturing and design of WT blades.
Aims to develop the preform manufacturing steps needed for Blade production: preform layup, consolidation, placement, and infusion. A virtual process chain will be developed to aid the optimization of the preform manufacturing processes to achieve defect-free WT blade manufacturing with low tolerances.
Recycled blade reinforcement materials and (re-)use in high performance products.
Will exploit the recyclable Recyclamine-based epoxy infusion resin codeveloped by SGRE as this has the greatest potential for increasing the circularity of WT blades in the near future.
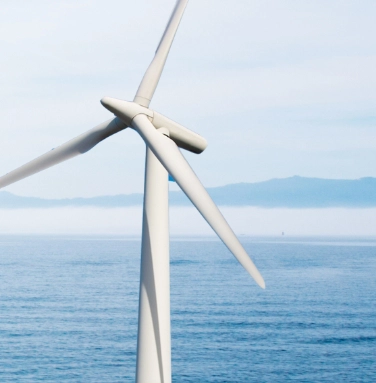
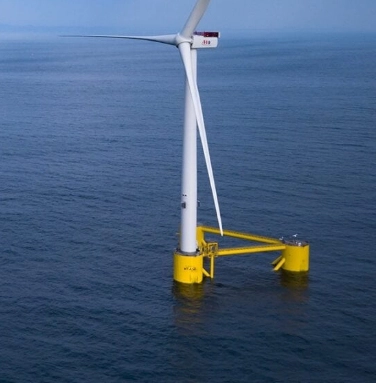
Lightweighted, circular and long-lasting solutions of floating WT structure
New materials for offshore floating WT supporting structures
ACCIONA’s CT-bos20 solution will be taken as a base case for MADE4WIND. The CT-bos is a TLP platform with a geometry based on a concrete port caisson which provides an easily adaptable solution for several turbine sizes without an overall impact on cost or size.
Aluminium rebar to replace steel for increased concrete durability
Will explore advanced modelling techniques, using and improving the constitutive models available in advanced commercial FE software such as Abaqus, in order to simulate Dynamic loads and fracture (e.g. the ‘concrete damaged plasticity’ 24 is a viable option). These constitutive models require a complex calibration procedure, and several mechanical tests, which will also be performed in MADE4WIND
Compact and more reliable WT drivetrain
Elimination of heavy rare earth magnets
A strategy to employ magnets with lower magnet properties i.e. less rare earth materials includes the following key aspects: reducing the power losses, through integrated optimized design of generator and converter; optimizing the demagnetization criteria which allows magnet with lower magnet properties to be utilized; and, the magnet topology optimization
Lightweighted drivetrains for FWT through compact and modular generator and converter
Will investigate the influence of the tower top motions on the drivetrain life.
Improved reliability of generator and converter
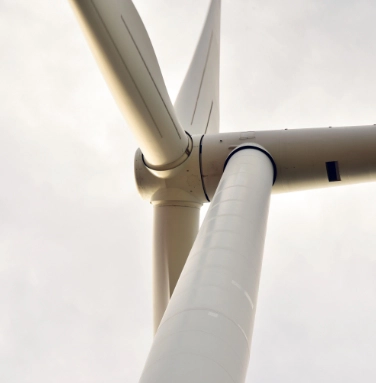
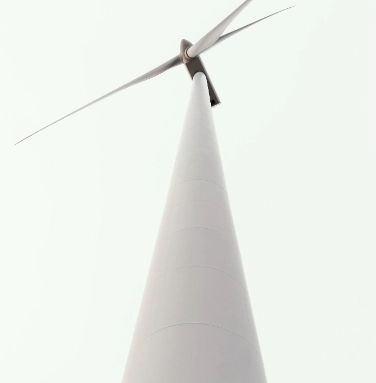
Development of the intelligent predictive computational modelling tools
Improved modelling tool for cost and LCoE analysis
Will develop an insulation of the bearing for the generator that will increase
the insulation resistance by factor of 3
Novel maintenance strategies and monitoring techniques
will develop and extend the work of RealTide and apply it to FWTs. Alongside this, novel techniques for electrical quantities will be developed by studying different potential analysis tools (e.g. Park’s vector method, the current signal method).
electrical quantities, studying different potential analysis tools (e.g. Park’s vector method, the current-signal method Generator air gap monitoring…).